Small defects within liquid crystals have the potential to host chemical reactions or to transport cargo, making them a potential candidate for exciting new technologies, like synthetic platforms for entirely new materials, drug delivery systems, and sensors.
Researchers at the University of Chicago’s Pritzker School of Molecular Engineering (PME) previously took a step toward these technologies by creating liquid crystals that could move autonomously.
Now, the same researchers have shown in simulations that they can precisely control the movement of defects within these active liquid crystals by changing the gradient of activity around them. In theory, this can be done by emitting pulses of light or changing the chemical composition in different areas of the system.
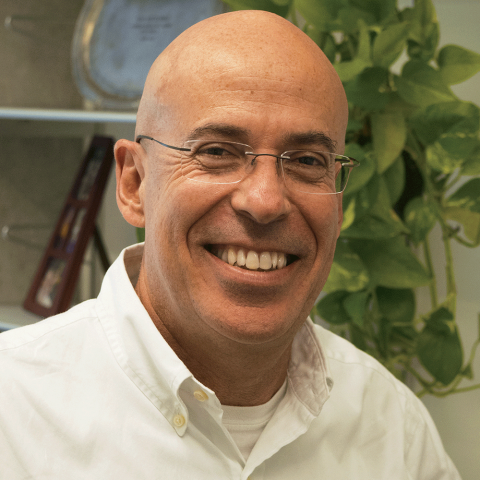
“Previously, the flows within these liquid crystal systems have been chaotic,” said Juan de Pablo, Liew Family Professor of Molecular Engineering, who led the research. “Now we have found a way to introduce gradients of activity within the system in a manner that confines and controls molecular motion, without having to use any barriers or obstacles.”
The results were published June 2 in Physical Review Letters. Other authors on the paper include postdoctoral researchers Rui Zhang, Ali Mozaffari, and Noe Atzin.
Controlling movements, creating patterns
In contrast to traditional liquids, liquid crystals exhibit a uniform molecular order. Such crystals have been used in optical technologies, like displays or communications, but they have the potential to be used in much more advanced technologies, such as capsules implanted in your body that can automatically release antibodies in response to a virus, or clothing that senses and captures toxic contaminants from the air.
One step toward creating such technologies is developing autonomous materials that can be controlled remotely. Previously, de Pablo and collaborators at UChicago and Stanford University developed autonomous liquid crystals by mixing in actin filaments — the same filaments that constitute a cell’s cytoskeleton — and “motor” proteins, the proteins that biological systems use to exert force on actin filaments. In this particular case, the proteins were engineered to be light-sensitive, which means their activity increases when exposed to light.