Understanding the behavior of matter at the level of molecules—how they bond, react, and change— is crucial for designing better materials, creating new medicines, and solving environmental challenges.
To understand these systems, researchers use quantum chemical computer simulations, which can interpret and predict new molecular systems and behavior, including catalytic processes, quantum phenomena, light-matter interactions.
Over the past 10 years, Prof. Laura Gagliardi of the University of Chicago Pritzker School of Molecular Engineering (UChicago PME) and her collaborator Prof. Don Truhlar at the University of Minnesota have developed and refined a theory that makes it feasible to study larger quantum systems.

Now, they have advanced that theory with a new method that achieves high accuracy without the steep computational cost of other advanced methods.
The results were published in the Proceedings of the National Academy of Sciences.
“We think this method could be a game-changer in terms of understanding complex chemistry and materials science problems,” said Gagliardi, the Richard and Kathy Leventhal Professor in UChicago PME and the Department of Chemistry. “It’s a way to study certain phenomena at a much more affordable computational cost.”
Building on a theoretical breakthrough
A method developed decades ago called the Kohn-Sham Density Functional Theory (KS-DFT) revolutionized the way scientists simulate and understand the quantum behavior of atoms, molecules, and materials. It balances accuracy and computational efficiency, making it one of the most widely used methods in quantum chemistry, condensed matter physics, and materials science.
Before KS-DFT, accurate quantum simulations required computationally expensive methods, such as wave function-based techniques. KS-DFT, however, achieves high accuracy for many systems at a fraction of the computational cost. This efficiency allows scientists to study large systems—like proteins, materials, or nanostructures—that would otherwise be inaccessible with traditional wave function methods.
But KS-DFT faces challenges with systems where electron interactions are complex and cannot be accurately described by a single-determinant wave function. These systems exhibit significant static correlation, meaning that multiple electronic configurations contribute substantially to their ground or excited states.
Examples include:
- Transition metal complexes
- Bond-breaking processes
- Molecules with near-degenerate electronic states
- Magnetic systems or excited states
Such systems are common in catalysis, photochemistry, and strongly correlated materials.
A new theory with lower computational cost
To find a way to better understand these systems, Gagliardi and Truhlar developed a novel form of density functional theory called multiconfiguration pair-density functional theory (MC-PDFT).
MC-PDFT offers more accuracy than advanced wave function methods but at a much lower computational cost. This makes it feasible to study larger systems that are prohibitively expensive for traditional wave-function methods.
MC-PDFT calculates the total energy by splitting it into two parts:
- The classical energy (kinetic energy, nuclear attraction, and Coulomb energy), which is obtained from the multiconfigurational wave function.
- The nonclassical energy (exchange-correlation energy), which is approximated using a density functional based on the electron density and the on-top pair density (a measure of the likelihood of finding two electrons close together).
This makes MC-PDFT a hybrid approach: it combines ideas from wave function theory and density functional theory to handle both weakly and strongly correlated systems.
But until now, MC-PDFT has relied on functional expressions that depended only on the density and its gradient, and the functional form had not been optimized.
Adding kinetic energy density for higher accuracy
To change that, Gagliardi and Truhlar created MC23, a new functional that depends on an additional ingredient: the kinetic energy density, which enables a more accurate description of electron correlation.
By fine-tuning the functional parameters using an extensive set of training systems—ranging from simple molecules to highly complex ones—Gagliardi and Truhlar created a tool that works well across the board of chemical complexity.
With MC23, researchers can now achieve high accuracy without the steep computational cost of other advanced methods. It also improves performance for spin splitting, bond energies, and multiconfigurational systems compared to previous MC-PDFT and KS-DFT functionals.
These innovations make MC23 a versatile and highly accurate functional for studying a wide variety of systems, particularly those where electron correlation effects are complex and cannot be captured by simpler methods.
2025 International Year of Quantum Science and Technology
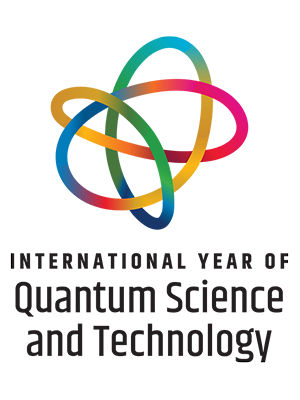
The United Nations declared 2025 the International Year of Quantum to mark a century of progress in quantum science and engineering. The University of Chicago and its partners join the celebration of the groundbreaking fields that continue to positively impact lives around the world.