The University of Chicago Pritzker School of Molecular Engineering (UChicago PME) is well-represented at the American Physical Society (APS) Global Physics Summit this week.
APS typically hosts separate March and April meetings, each dedicated to a different area of the physical sciences. For 2025, they combined the meetings to create an interdisciplinary event where research and researchers break free of traditional academic silos.
It's an area where PME - UChicago's interdisciplinary engineering school - quite naturally excels.
With more than 14,000 researchers gathering in Anaheim, California, through Friday, here are a few more ways UChicago PME is impacting the global scientific community.
UChicago PME presentation highlights
Engineering a quantum future for societal impact
David Awschalom, Liew Family Professor of Molecular Engineering and Vice Dean for Research and Infrastructure; Director, Chicago Quantum Exchange; Director, Q-NEXT
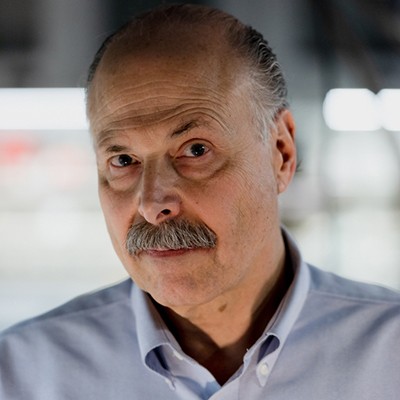
The rapidly advancing field of quantum technology has the potential to reshape our society in the coming decades, in ways we can predict - and in ways we cannot. It could bring us transformative innovations across industries: quantum sensors could be used to identify disease at its smallest and earliest stages, pilot planes with more accurate and reliable navigation, and improve environmental monitoring. Quantum networks could increase the security of financial networks, even enhance democracy by enabling provably secure voting systems. Fault-tolerant quantum computers could accelerate the discovery of life-saving drugs, pinpoint fraud through the quick analysis of vast datasets, optimize transportation systems and construction schedules, enable the design of new materials with specific properties, and create a more resilient energy grid. This revolution could bring hundreds of thousands of jobs for people from a wide variety of backgrounds and education levels and create billions of dollars in economic impact by 2035. To reap these benefits, however, we need to begin thinking in new ways to enable significant technical advances and coordination to enable societal impact. These include building models of open and rigorous training to match workforce demand; creating more integrated collaboration mechanisms among academic, government, and industry stakeholders to accelerate commercialization and education efforts; and adopting government policies that balance the essential role of international cooperation with the need to protect intellectual property. These preparations and flexibility in the face of uncertainty are particularly crucial because, as past technology transformations have shown us, some of the biggest and most exciting societal impacts may well be the ones we have not yet imagined.
A modular approach to superconducting quantum information processing
Andrew Cleland, John A. MacLean Sr. Professor of Molecular Engineering Innovation and Enterprise; Director, Pritzker Nanofabrication Facility
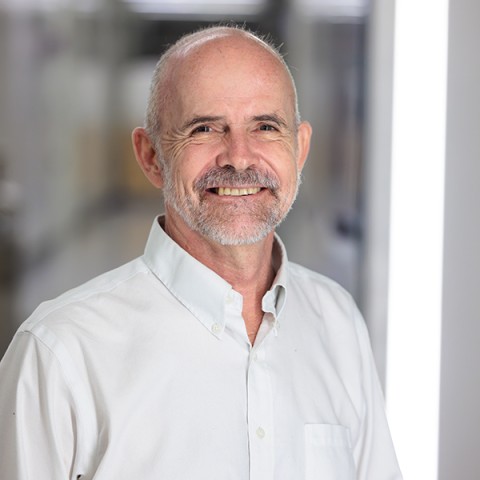
Modular architectures for quantum computing present an interesting alternative to the popular monolithic approach. This is especially true for solid-state based computing such as with superconducting qubits: Assembling a quantum processor from small pre-selected modules promises higher average performance qubits with fewer drop-outs, as well as offering the opportunity to shield modules from one another, reducing cross-talk and increasing the available frequency span within each module. Protection from cosmic-ray-like events may also be possible. Challenges in modular architectures include designing circuits that can accommodate the unavoidably smaller interconnect densities between modules, with the concomitant challenge of preserving error-protection functionality. In this talk I will describe our approach to a modular superconducting quantum processor, using an assembly of separate qubit daughterboards interconnected via a router-supported motherboard, in which externally controlled routers selectively couple qubits on daughterboards flip-chip coupled to the motherboard. The routers support multi-qubit entangling operations, controlled using model-free reinforcement learning, including two-qubit controlled-Z gates as well as three-qubit controlled-swap gates. If time permits, I will also describe recent measurements of cosmic-ray-like events in these assemblies.
Non-Gaussian generalized two-mode squeezing: applications to two-ensemble spin squeezing and beyond
Aashish Clerk, Professor of Molecular Engineering
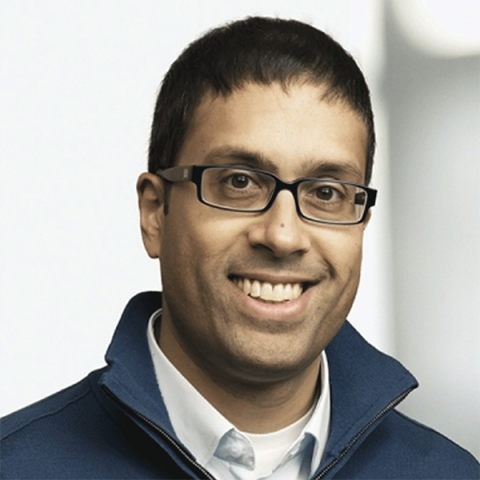
Bosonic two-mode squeezed states are paradigmatic entangled Gaussian states that have wide utility in quantum information and metrology. Here, we show that the basic structure of these states can be generalized to arbitrary bipartite quantum systems in a manner that allows simultaneous, Heisenberg-limited estimation of two independent parameters for finite-dimensional systems. Further, we show that these general states can always be stabilized by a relatively simple Markovian dissipative process. In the specific case where the two subsystems are ensembles of two-level atoms or spins, our generalized states define a notion of two-mode spin squeezing that is valid beyond the Gaussian limit and that enables true multi-parameter estimation. We discuss how generalized Ramsey measurements allow one to reach the two-parameter quantum Cramer-Rao bound, and how the dissipative preparation scheme is compatible with current experiments.
Integrated quantum technologies with diamond membranes
Alex High, Assistant Professor of Molecular Engineering
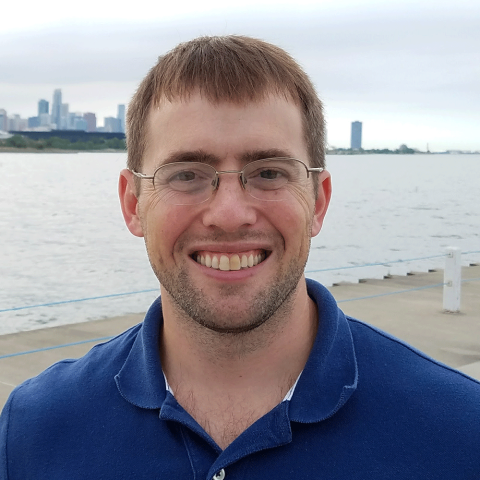
The continued evolution of quantum technologies in diamond requires heterogenous material platforms for sophisticated functionalities, device integration and packaging, and improved qubit performance. At UChicago and Argonne National Laboratory, we are creating pristine single-crystal diamond membranes that host coherent color center qubits and integrating them with a wide range of materials including fused silica, sapphire, thermal oxide, lithium niobate, tantalum, silicon and YIG. The membrane uniformity and robustness to fabrication enables sophisticated device fabrication. We demonstrate several varieties of integrated nanophotonic cavities – critical elements in quantum photonics - with record quality factors. Furthermore, we show that our ultra-thin diamond membranes are compatible with total internal reflection fluorescence (TIRF) microscopy, which enables interfacing coherent diamond quantum sensors with living cells while rejecting unwanted background luminescence. Remarkably, we demonstrate that membrane integration can improve the spin coherence and microwave addressability of tin vacancy qubits while maintaining exceptional optical coherence, allowing us to create an operational spin-photon interface at 4 Kelvin. In total, the combination of qubit performance, high-performance device fabrication, and flexible materials integration will enable a broad range of quantum photonic, acoustic, and sensing science and technologies.
Membranes for Redox Flow Batteries
Shrayesh Patel, Associate Professor of Molecular Engineering
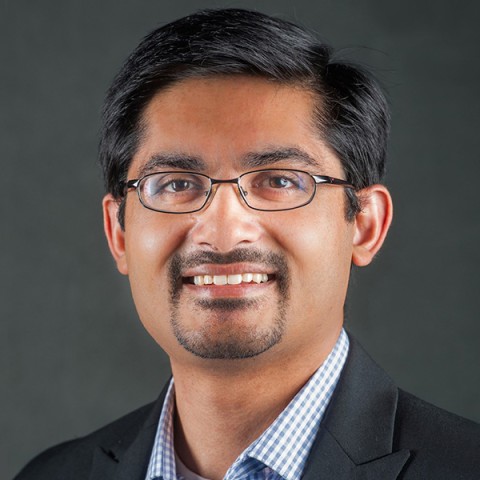
Membranes are one critical component in redox flow batteries (RFBs), separating active species and allowing ions transfer. These membranes not only could dominate the cycling performance of RFBs, but also impact the overall system cost as they are usually very expensive due to their complex manufacturing. By adopting an efficient non-solvent phase separation (NIPS) method and a tunable post-sulfonation process, we have successfully used a low-cost industrial-scale plastic, blend of poly[phenylene oxide] and high impact polystyrene, into porous ion exchange membranes tailored to aqueous RFB systems. The fabricated membranes shows durable interconnected mesopores with fine-tuned sulfonation, resulting in low area-specific resistance (ASR) and excellent permeability in both acidic and alkaline electrolytes. Flow cell characterization using either 2,6-dihydroxyanthraquinone (2,6-DHAQ) / K4Fe(CN)6 or vanadium salts indicates such membranes can deliver outstanding capacity retention and power density. This work exemplifies the approach of using low-cost plastics into valuable products, representing an exciting and sustainable opportunity for addressing critical challenges for long duration energy storage solutions.
Q-NEXT: Advancing Science and Technology for a Quantum Interconnected Future
David Awschalom, Liew Family Professor of Molecular Engineering and Vice Dean for Research and Infrastructure; Director, Chicago Quantum Exchange; Director, Q-NEXT
Q-NEXT is developing the science and technology for controlling, storing, and transmitting quantum information for breakthroughs in fundamentally secure communications, networks of ultraprecise sensors, and interconnecting future quantum computers. In its four years of operation, the Q-NEXT center has launched two national quantum foundries and used them to populate a materials and devices database, demonstrated novel and high-performance qubit platforms, installed a quantum computing testbed, and developed the science and technology that will help enable scalable quantum networks. Q-NEXT has collaborated across industry, academia and national laboratories toward the creation of a roadmap to create impact from quantum interconnect technology. [1] The Center has established itself at the forefront of the national quantum ecosystem through unique research experiences for students, educational tools for teachers, and by engaging the public through popular quantum-themed events. As it looks ahead, Q-NEXT will draw on its foundation of scientific results and physical facilities to reinforce a domestic supply of quantum materials and devices for research and to accelerate quantum science and technology intro practice through collaboration with established and emerging companies - all toward national leadership in this critically important field.