Researchers from across disciplines and the globe gathered in Seattle last week to provide a window into the future of materials science.
UChicago Pritzker School of Molecular Engineering (UChicago PME) was at the 2025 Materials Research Society Spring Meeting & Exhibit in force, sharing insights on energy storage, polymers, bioelectronics, quantum innovations and other ways materials can make a better world.
Here are just a few of the ways UChicago PME’s thought leadership is driving change on a global level:
UChicago PME presentation highlights
Quantum Spintronics with Silicon Carbide and Oxides
David Awschalom, Liew Family Professor of Molecular Engineering and Vice Dean for Research and Infrastructure; Director, Chicago Quantum Exchange; Director, Q-NEXT
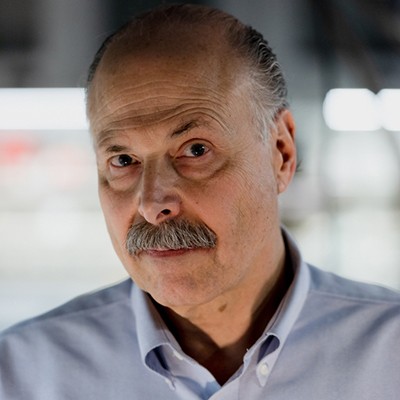
Optically active spin defects in semiconductors can be used as quantum bits for information processing and sensing technologies. These spin qubits have a built-in spin-photon interface that emits in the visible, infrared, and telecom bands which is crucial for quantum networks, retain their quantum properties over millisecond timescales or longer, can be manipulated using a simple combination of light and microwave pulses and can be integrated into electronically active devices. We discuss recent advances in this area including the integration of single spin qubits into silicon carbide (SiC) devices, as well as oxides, and pathways to extend spin coherence times.
SiC offers a powerful technological platform, mature device integration, and broad compatibility with CMOS fabrication techniques. In particular, the neutral divacancy complex (VV0) has been isolated in SiC optoelectronic devices with lifetime limited single-photon emission and enables strategies for addressing charge noise sensitivity. We can further improve the fundamental quantum properties of these defects through isotopic engineering of the local nuclear spin environment. Combining all these elements make divacancy defects in SiC an ideal platform for integrated devices, with access to both excellent optical properties and long-lived nuclear spin memories.
In addition to exploring the physics of the neutral divacancy (VV0), we are engineering new defect-based spin qubits in SiC for quantum communication and networks. Transition metal ions such as vanadium have host-agnostic orbital structures and operate in the (near) telecom regime, and therefore could be naturally integrated into pre-existing telecommunications networks without the need for complicated frequency conversion schemes. With decreasing temperature, we observe a remarkable four-orders-of-magnitude increase in spin relaxation time and identify the underlying relaxation mechanisms which involve a two-phonon Orbach process. These results position vanadium as a prime candidate for scalable quantum nodes in future quantum networks.
Integrating these findings, we present a qubit system Er3+: CeO2 (cerium dioxide) in a nuclear spin free environment for supporting long-lived spins with a telecom C-band spin-photon interface for applications in fiber-based quantum networks. We describe the electron spin coherence of Er3+ ions doped in CeO2 epitaxial films grown on Si substrates. The reduced nuclear spin noise in the host yields an electron spin coherence of 0.66 μs in the isolated ion limit and a spin relaxation of 2.5 ms, all measured at T=3.6 K. These results indicate the potential of this system as a flexible platform for quantum communication.
Heterogeneous Integration of Solid State Defect and Dopant Qubit Systems on Silicon
Supratik Guha, Professor of Molecular Engineering; Senior Advisor to Argonne Physical Sciences and Engineering
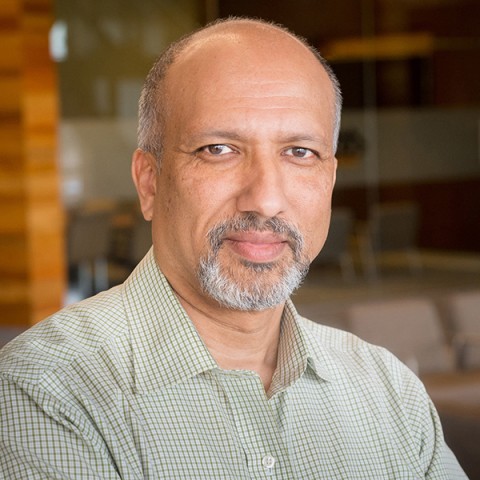
Solid state defect or dopant qubits embedded in wide gap semiconductor and insulator hosts are important for devices in quantum information processing such as sensors, quantum memories and single photon emitters. Many of these materials belong to the “exotic” to “expensive” class (e.g. diamond, SiC, YIG, various rare earth and other oxides): they can be difficult to process, ,or are limited in availability and purity. In addition to meeting performance specifications, turning these compounds into members of the “electronics materials proletariat”--making them chip-scale, on Si platforms, processable using scalable cleanroom techniques, and integrable with electronics--is the challenge. With this in mind, we will describe our work in two ongoing directions.
The Er atom offers a unique spin-photon interface compatible with long-distance optical fiber communications due to its electron spin qubit combined with its 1.5 mm (telecom C-band) optical transition. This has led to broad effort on establishing Er-doped oxide host systems as quantum memory devices, a limiting component for the quantum repeaters necessary for long distance quantum communication. Our work has targeted developing such Er-doped metal oxide thin films (TiO2, CeO2, CaMoO4, Y2O3) on Si substrates. Correlating optical (inhomogeneous & homogeneous linewidths, spectral diffusion via photoluminescence excitation) and microstructural (electron microscopy and X-ray diffraction) analysis with growth studies that explore crystallinity, Er density, and film thickness across a large number of samples and different materials, we have explored the factors that control optical properties – for example, showing evidence of the role of charged defects and Er density variation. We have further explored processing and nanofabrication of 1-D photonic cavities demonstrating Purcell enhancement of ~300X in Er:TiO2/Si heterostructures, the isolation of emission from single Er+3 ions, and the adaptation of atomic layer deposition (ALD) techniques for ppm doped Er:TiO2 and Er: CeO2.
We are also studying the heterogeneous integration of materials by “spalling” 1-50 mm thick large area layers from single crystal wafers of materials relevant to quantum technologies such as SiC, diamond and YIG/GGG and integrating them onto arbitrary platforms without the need for high thermal budgets or ion implantation. While spalling of low fracture toughness Si and GaAs is well established, the spalling of significantly harder materials relevant for us is new and we will describe our design of such spalling methods. We have spalled commercially available high purity 4H-SiC and achieve coherent spin control of neutral divacancy (VV0) qubit ensembles with quasi-bulk spin coherence in the exfoliated films.
Integrated Quantum Technologies with Diamond Membranes
Alex High, Assistant Professor of Molecular Engineering
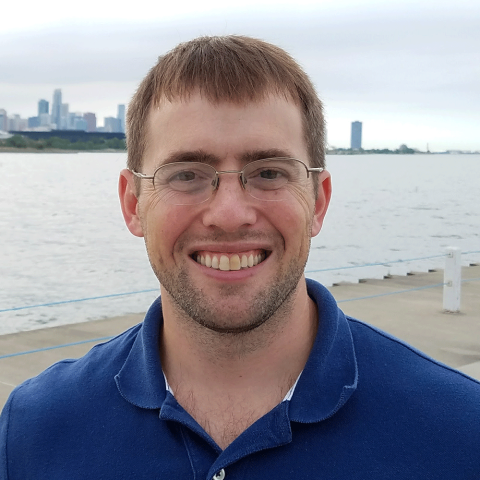
The continued evolution of quantum technologies in diamond requires heterogenous material platforms for sophisticated functionalities, device integration and packaging, and improved qubit performance. At UChicago and Argonne National Laboratory, we are creating pristine single-crystal diamond membranes that host coherent color center qubits and integrating them with a wide range of materials including fused silica, sapphire, thermal oxide, lithium niobate, tantalum, silicon and YIG. The membrane uniformity and robustness to fabrication enables sophisticated device fabrication. We demonstrate several varieties of integrated nanophotonic cavities – critical elements in quantum photonics - with record quality factors. Furthermore, we show that our ultra-thin diamond membranes are compatible with total internal reflection fluorescence (TIRF) microscopy, which enables interfacing coherent diamond quantum sensors with living cells while rejecting unwanted background luminescence. Remarkably, we demonstrate that membrane integration can improve the spin coherence and microwave addressability of tin vacancy qubits while maintaining exceptional optical coherence, allowing us to create an operational spin-photon interface at 4 Kelvin. In total, the combination of qubit performance, high-performance device fabrication, and flexible materials integration will enable a broad range of quantum photonic, acoustic, and sensing science and technologies.
Dynamic Radiative Thermoregulation for Human-Building-Energy Nexus
Po-Chun Hsu, Assistant Professor of Molecular Engineering
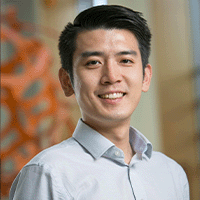
The human body strives to maintain the body temperature for proper physiological functions. The invention of heating and air conditioning drastically increases humans’ adaptability to thermal environments, but it also results in significant energy consumption and greenhouse gas emissions. Radiative thermoregulation has immense untapped potential in expanding the controllable heat transfer pathways and tunable range that can benefit both personal thermal comfort and building energy efficiency in a wide variety of climate zones and user conditions. In this talk, I will introduce our recent work in dynamic thermoregulation for both human body and building envelope. The triggers can be mechanical force, moisture-swelling, and electrochemical potentials, each with unique multiphysics design principles and advantages.
Mid-Infrared Electrochromics by High Conductivity Conjugated Polymers
Po-Chun Hsu
Mid-infrared (MIR) electrochromics uses electrochemical potentials to control the radiative heat transfer in a non-volatile and efficient manner, holding great potential for thermoregulation, a crucial technology in the era of global warming and climate change. The working principle is to use redox reactions to vary the charge carrier concentration to affect the light-matter interaction. In this talk, I will introduce a series of work of MIR electrochromics based on conjugated polymers. We suggest that a good MIR electrochromic polymer should simultaneously have high electrical and ionic conductivity, a requirement that is similar to organic mixed ion-electron conductors (OMIEC) but with more emphasis on the metallicity rather than semiconducting. High electrical conductivity ensures the low emissivity state, and high ionic conductivity allows the polymer to be switched to the high emissivity state via redox reaction. I will introduce MIR electrochromics examples using PEDOT:PSS and polyaniline and how structure-property relationship study helps us understand the mechanism for further molecular engineering.
Interfacing Biomolecules with Coherent Quantum Sensors
Peter Maurer, Assistant Professor of Molecular Engineering
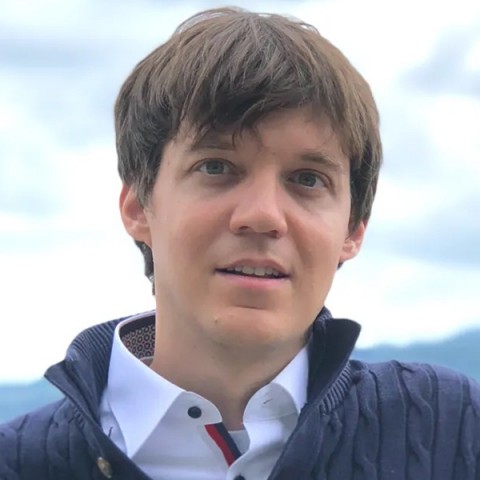
Quantum metrology enables some of the world's most sensitive measurements with potentially far-reaching applications in the life sciences. Although the ultrahigh sensitivity of qubit sensors has spurred the imagination of researchers, implementation in actual devices that enable monitoring cellular processes or detecting diseases still remains largely elusive. Overcoming limitations that hold back wider application of quantum technology in the life sciences, requires advances in both fundamental science and engineering. In this talk, I will discuss our research group's recent results on addressing one of these long-standing research challenges, namely, how to interface highly coherent quantum sensors with biological target systems. My discussion will start with the development of a novel biocompatible surface functionalization architecture for highly coherent diamond crystals. I will then continue with discussing a new approach to engineering spin coherence in core-shell structured diamond particles, which can be readily chemically modified and delivered to intact biological systems. Finally, I will conclude my talk with an outlook on a novel class of molecular qubit sensors that will overcome many of the fundamental challenges associated with current diamond-based quantum sensors. The unifying theme of these advances are the convergence of techniques from single-molecule biophysics, material science, and quantum engineering. Specific applications of the developed sensing platforms to questions in the life sciences will be discussed throughout this talk.
Bioadhesive and Immune-Compatible Polymer Bioelectronics
Sihong Wang, Assistant Professor of Molecular Engineering
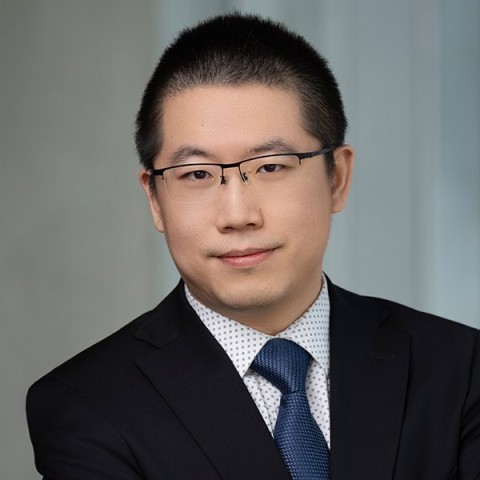
Implantable bioelectronics have prevalent applications in fundamental biological studies, disease diagnosis, and medical treatment. To ensure high-quality signal transductions, the interfaces between bioelectronic devices and bio-tissues must have stable and conformable contact. However, this remains a major challenge due to several reasons. First, mediated by the immune system, implantable devices all suffer from the excessive ingrowth of collagen and inflammation reactions around the device, which is known as the foreign-body response (FBR). The fibrosis layer formed at the device-tissue interface leads to a substantial increase in the impedance and thereby limits the longevity of the device. Second, the lack of tissue/skin adhesion properties on electronic materials (i.e., conductors and semiconductors) prevents the formation of intimate and long-term stable bio-interfaces. The research in my group for addressing these challenges has been focusing on electronic polymers, which have the best biocompatibility and broad design tunability. In this presentation, I will introduce a set of molecular design strategies for enhancing the immune compatibility of semiconducting polymers. I will also introduce our material and device designs for introducing tissue-adhesive properties onto polymer semiconductors and transistor-based biosensors. Then, I will show the strategies and advantages of using these new biomimetic properties in bioelectrical and biochemical sensing.
Sihong Wang
The vast amount of biological mysteries and biomedical challenges faced by humans provide a prominent drive for seamlessly merging electronics with biological living systems (e.g. human bodies) to achieve long-term stable functions. Towards this trend, one of the key requirements for electronics is to possess biomimetic form factors in various aspects for achieving long-term biocompatibility. To enable such paradigm-shifting requirements, polymer-based electronics are uniquely promising for combining advanced electronic functionalities with biomimetic properties. Among all the functional materials, stretchable light-emitting materials are the key components for realizing skin-like displays and optical bio-stimulation. In this talk, I will mainly introduce our research in imparting stretchability onto “third-generation” electroluminescent polymers that can harness all the excitons through thermally activated delayed fluorescence (TADF), thereby with a theoretical near-unity quantum yield and high OLED efficiency. Our developments of fully stretchable OLED devices show the promise of achieving all the desired EL and mechanical characteristics, including high efficiency, brightness, switching speed, stretchability, and low driving voltage.